To precisely understand the status of scattered strontium-90 (90Sr) after the 2011 accident at the Fukushima Daiichi Nuclear Power Plant (F1-NPP) of Tokyo Electric Power Company (TEPCO), the measurement of the soil samples collected both before and after the day of the accident from the same sampling locations is necessary. However, very few reports have investigated the background contaminant data before the accident even though several studies have been conducted to investigate the effects of the F1-NPP accident. To address the lack of the passed 90Sr information and reestablished baseline, this study focuses on the stored topsoil samples that are collected from the same sampling locations from the Fukushima Prefecture before and after the F1-NPP accident, which are analyzed for obtaining the 90Sr concentrations. The results of our investigation exhibited that the 90Sr concentrations in the Fukushima Prefecture soils ranged from 0.2 to 20.4 Bq/kg in the samples that were collected before the accident and from 1.37 to 80.8 Bq/kg in the samples that were collected after the accident from identical sampling locations. Further, the soil samples that were collected from 30 out of 56 locations displayed significant differences in terms of concentrations before and after the accident. In addition, the relations between the 90Sr concentrations and the soil properties of the samples (organic content, pH, water content, and composition) were investigated, and it was found that the organic content and water content had a positive correlation with 90Sr concentrations and, in contrast, the sandiness was shown to have a negative correlation with 90Sr concentrations. The depth characteristics were also investigated. The aforementioned results indicate that this tendency would be observed even in the future.
A large amount of radioactive materials was scattered throughout the environment (ocean, atmosphere, land, and so on) because of the accident that occurred on March 11, 2011 at the Fukushima Daiichi Nuclear Power Plant (F1-NPP) that was owned by Tokyo Electric Power Company Holdings, Inc. (TEPCO).(1−3) Seven years have passed by since the accident, and research institutes around the world have been monitoring the influence of the environmental dynamics of radionuclides that have been released.(4−13) More specifically, there have been several environmental monitoring reports regarding β-ray-emitting nuclides, such as radioiodine and radiocesium, because multiple samples can be analyzed in a relatively short time using certain types of instruments such as a germanium semiconductor detector, a sodium iodide scintillator detector, and a lantern bromide scintillator detector.(14−19) Meanwhile, radiostrontium (90Sr) (half-life: 28.79 y(20)) is a pure β-ray-emitting nuclide that does not emit γ-rays, which makes it necessary to chemically isolate it for measuring β-rays because the β-ray spectra overlap. In particular, it is imperative to monitor 90Sr over a long period because it will require several decades to decommission F1-NPP. In Japan, instead of a few literature concerning the development of a rapid analytical means,(21−25) radiochemical analysis using milking-low background gas-flow counter (milking-LBC) is adopted as the official analysis method for analyzing 90Sr because of good sensitivity and/or high-precision analysis in low concentration levels in the environment.(26) This method requires considerable amount of time and effort to pretreat the analysis as compared to those required by the γ-ray measurement method. Although various studies have been vigorously conducted,(27−33) the study related to the scattering of 90Sr is not as advanced as compared to that related to the γ-ray-emitting nuclides such as radiocesium.
To precisely understand the status of scattered 90Sr after an incident of nuclear accident, the samples collected both before and after the day of the accident should be measured, thereby distinguishing from the fallout of atmospheric nuclear tests (20th century’s) that have been conducted in the past. So far, the survival ratios of nuclides with short half-lives in samples have been employed in several studies.(34) However, this technique cannot track the long-term process because it becomes difficult to evaluate the nuclides that exhibit a short decrease in half-lives. The optimal method for addressing these issues is to measure the radioactive concentrations of 90Sr in soil that is collected at identical locations before and after the accident. However, few examples exhibited the presence of 90Sr in soil before the F1-NPP accident, which was completely unexpected. Fortunately, we already possessed analytical data related to the 90Sr concentrations in soil samples that were collected before the accident with precise sampling locations throughout the Fukushima Prefecture (not published). Therefore, in this study, we succeeded in estimating the exact amount of 90Sr deposition before and after the F1-NPP accident. When performing the long-term observation, understanding the background level of 90Sr before the accident was observed to be considerably important for understanding the environmental radioactivity and the environmental dynamics or the usage of 90Sr as a tracer.
In this study, we measured the radioactivity concentrations of 90Sr in the topsoil at the same locations in the Fukushima Prefecture before and after the accident and obtained the background levels of 90Sr before the F1-NPP accident. Thus, we revealed the deposition status of 90Sr before and after the accident. We also investigated the correlation between the soil properties and 90Sr to determine the status of deposition of 90Sr on the topsoil in Fukushima prefecture (Figure 1).
December 27, 2018
Posted by dunrenard |
Fukushima 2018 | Contamination, Fukushima, Strontium-90, Topsoil |
Leave a comment
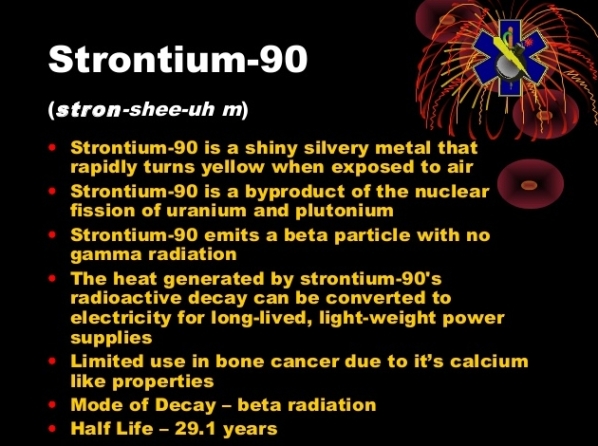
Highlights
- •
-
90Sr in cattle teeth was determined after the Fukushima accident.
- •
-
90Sr was efficiently incorporated into the teeth at development stages.
- •
-
90Sr specific activity of aqueous extract was higher than that of soil.
- •
-
Water soluble 90Sr was incorporated into the cattle and accumulated.
- •
-
Sr transfer is eliminated compared to Ca in the migration route.
Abstract
90Sr specific activity in the teeth of young cattle that were abandoned in Kawauchi village and Okuma town located in the former evacuation areas of the Fukushima-Daiichi Nuclear Power Plant (FNPP) accident were measured. Additionally, specific activity in contaminated surface soils sampled from the same area was measured. (1) All cattle teeth examined were contaminated with 90Sr. The specific activity, however, varied depending on the developmental stage of the teeth during the FNPP accident; teeth that had started development before the accident exhibited comparatively lower values, while teeth developed mainly after the accident showed higher values. (2) Values of 90Sr-specific activity in teeth formed after the FNPP accident were higher than those of the bulk soil but similar to those in the exchangeable fraction (water and CH3COONH4 soluble fractions) of the soil. The findings suggest that 90Sr was incorporated into the teeth during the process of development, and that 90Sr in the soluble and/or leachable fractions of the soil might migrate into teeth and contribute to the amount of 90Sr in the teeth. Thus, the concentration of 90Sr in teeth formed after the FNPP accident might reflect the extent of 90Sr pollution in the environment.
http://www.sciencedirect.com/science/article/pii/S0265931X17307610
December 23, 2017
Posted by dunrenard |
Fukushima 2017 | Cattle teeth, Fukushima Radiation, Strontium-90 |
Leave a comment
TEPCO released a document stating that 30Bq/kg of Strontium 90 was found in sea breams in the sea 2km from the Kido river (near J-Village).
Click to access fish03_170713-j.pdf



July 22, 2017
Posted by dunrenard |
Fukushima 2017 | Fukushima, Sea Breams, Strontium-90 |
Leave a comment

With specific information on Tritium, Strontium 90, Cesium 137, radioactive Iodine 131, and Plutonium.
By Helen Caldicott, Volume 4, Issue 2 2014, Australian Medical Student Journal
…Fukushima is now described as the greatest industrial accident in history.
The Japanese government was so concerned that they were considering plans to evacuate 35 million people from Tokyo, as other reactors including Fukushima Daiini on the east coast were also at risk. Thousands of people fleeing from the smoldering reactors were not notified where the radioactive plumes were travelling, despite the fact that there was a system in place to track the plumes. As a result, people fled directly into regions with the highest radiation concentrations, where they were exposed to high levels of whole-body external gamma radiation being emitted by the radioactive elements, inhaling radioactive air and swallowing radioactive elements. [2] Unfortunately, inert potassium iodide was not supplied, which would have blocked the uptake of radioactive iodine by their thyroid glands, except in the town of Miharu. Prophylactic iodine was eventually distributed to the staff of Fukushima Medical University in the days after the accident, after extremely high levels of radioactive iodine – 1.9 million becquerels/kg were found in leafy vegetables near the University. [3] Iodine contamination was widespread in leafy vegetables and milk, whilst other isotopic contamination from substances such as caesium is widespread in vegetables, fruit, meat, milk, rice and tea in many areas of Japan. [4]
The Fukushima meltdown disaster is not over and will never end. The radioactive fallout which remains toxic for hundreds to thousands of years covers large swathes of Japan and will never be “cleaned up.” It will contaminate food, humans and animals virtually forever. I predict that the three reactors which experienced total meltdowns will never be dissembled or decommissioned. TEPCO (Tokyo Electric Power Company) – says it will take at least 30 to 40 years and the International Atomic Energy Agency predicts at least 40 years before they can make any progress because of the extremely high levels of radiation at these damaged reactors.
This accident is enormous in its medical implications. It will induce an epidemic of cancer as people inhale the radioactive elements, eat radioactive food and drink radioactive beverages. In 1986, a single meltdown and explosion at Chernobyl covered 40% of the European land mass with radioactive elements. Already, according to a 2009 report published by the New York Academy of Sciences, over one million people have already perished as a direct result of this catastrophe. This is just the tip of the iceberg, because large parts of Europe and the food grown there will remain radioactive for hundreds of years. [5]
Medical Implications of Radiation
Fact number one
No dose of radiation is safe. Each dose received by the body is cumulative and adds to the risk of developing malignancy or genetic disease.
Fact number two
Children are ten to twenty times more vulnerable to the carcinogenic effects of radiation than adults. Females tend to be more sensitive compared to males, whilst foetuses and immuno-compromised patients are also extremely sensitive.
Fact number three
High doses of radiation received from a nuclear meltdown or from a nuclear weapon explosion can cause acute radiation sickness, with alopecia, severe nausea, diarrhea and thrombocytopenia. Reports of such illnesses, particularly in children, appeared within the first few months after the Fukushima accident.
Fact number four
Ionizing radiation from radioactive elements and radiation emitted from X-ray machines and CT scanners can be carcinogenic. The latent period of carcinogenesis for leukemia is 5-10 years and solid cancers 15-80 years. It has been shown that all modes of cancer can be induced by radiation, as well as over 6000 genetic diseases now described in the medical literature.
But, as we increase the level of background radiation in our environment from medical procedures, X-ray scanning machines at airports, or radioactive materials continually escaping from nuclear reactors and nuclear waste dumps, we will inevitably increase the incidence of cancer as well as the incidence of genetic disease in future generations.
Types of ionizing radiation
- X-rays are electromagnetic, and cause mutations the instant they pass through the body.
- Similarly, gamma radiation is also electromagnetic, being emitted by radioactive materials generated in nuclear reactors and from some naturally occurring radioactive elements in the soil.
- Alpha radiation is particulate and is composed of two protons and two neutrons emitted from uranium atoms and other dangerous elements generated in reactors (such as plutonium, americium, curium, einsteinium, etc – all which are known as alpha emitters and have an atomic weight greater than uranium). Alpha particles travel a very short distance in the human body. They cannot penetrate the layers of dead skin in the epidermis to damage living skin cells. But when these radioactive elements enter the lung, liver, bone or other organs, they transfer a large dose of radiation over a long period of time to a very small volume of cells. Most of these cells are killed; however, some on the edge of the radiation field remain viable to be mutated, and cancer may later develop. Alpha emitters are among the most carcinogenic materials known.
- Beta radiation, like alpha radiation, is also particulate. It is a charged electron emitted from radioactive elements such as strontium 90, cesium 137 and iodine 131. The beta particle is light in mass, travels further than an alpha particle and is also mutagenic.
- Neutron radiation is released during the fission process in a reactor or a bomb. Reactor 1 at Fukushima has been periodically emitting neutron radiation as sections of the molten core become intermittently critical. Neutrons are large radioactive particles that travel many kilometers, and they pass through everything including concrete and steel. There is no way to hide from them and they are extremely mutagenic.
So, let’s describe just five of the radioactive elements that are continually being released into the air and water at Fukushima. Remember, though, there are over 200 such elements each with its own half-life, biological characteristic and pathway in the food chain and the human body. Most have never had their biological pathways examined. They are invisible, tasteless and odourless. When the cancer manifests it is impossible to determine its aetiology, but there is a large body of literature proving that radiation causes cancer, including the data from Hiroshima and Nagasaki.
- Tritium is radioactive hydrogen H3 and there is no way to separate tritium from contaminated water as it combines with oxygen to form H3O. There is no material that can prevent the escape of tritium except gold, so all reactors continuously emit tritium into the air and cooling water as they operate. It concentrates in aquatic organisms, including algae, seaweed, crustaceans and fish, and also in terrestrial food. Like all radioactive elements, it is tasteless, odorless and invisible, and will therefore inevitably be ingested in food, including seafood, for many decades. It passes unhindered through the skin if a person is immersed in fog containing tritiated water near a reactor, and also enters the body via inhalation and ingestion. It causes brain tumors, birth deformities and cancers of many organs.
- Cesium 137 is a beta and gamma emitter with a half-life of 30 years. That means in 30 years only half of its radioactive energy has decayed, so it is detectable as a radioactive hazard for over 300 years. Cesium, like all radioactive elements, bio-concentrates at each level of the food chain. The human body stands atop the food chain. As an analogue of potassium, cesium becomes ubiquitous in all cells. It concentrates in the myocardium where it induces cardiac irregularities, and in the endocrine organs where it can cause diabetes, hypothyroidism and thyroid cancer. It can also induce brain cancer, rhabdomyosarcomas, ovarian or testicular cancer and genetic disease.
- Strontium 90 is a high-energy beta emitter with a half-life of 28 years. As a calcium analogue, it is a bone-seeker. It concentrates in the food chain, specifically milk (including breast milk), and is laid down in bones and teeth in the human body. It can lead to carcinomas of the bone and leukaemia.
- Radioactive iodine 131 is a beta and gamma emitter. It has a half-life of eight days and is hazardous for ten weeks. It bio-concentrates in the food chain, in vegetables and milk, then in the the human thyroid gland where it is a potent carcinogen, inducing thyroid disease and/or thyroid cancer. It is important to note that of 174,376 children under the age of 18 that have been examined by thyroid ultrasound in the Fukushima Prefecture, 12 have been definitively diagnosed with thyroid cancer and 15 more are suspected to have the disease. Almost 200,000 more children are yet to be examined. Of these 174,367 children, 43.2% have either thyroid cysts and/or nodules.In Chernobyl, thyroid cancers were not diagnosed until four years post-accident. This early presentation indicates that these Japanese children almost certainly received a high dose of radioactive iodine. High doses of other radioactive elements released during the meltdowns were received by the exposed population so the rate of cancer is almost certain to rise.
- Plutonium, one of the most deadly radioactive substances, is an alpha emitter. It is highly toxic, and one millionth of a gram will induce cancer if inhaled into the lung. As an iron analogue, it combines with transferrin. It causes liver cancer, bone cancer, leukemia, or multiple myeloma. It concentrates in the testicles and ovaries where it can induce testicular or ovarian cancer, or genetic diseases in future generations. It also crosses the placenta where it is teratogenic, like thalidomide. There are medical homes near Chernobyl full of grossly deformed children, the deformities of which have never before been seen in the history of medicine.The half-life of plutonium is 24,400 years, and thus it is radioactive for 250,000 years. It will induce cancers, congenital deformities, and genetic diseases for virtually the rest of time.Plutonium is also fuel for atomic bombs. Five kilos is fuel for a weapon which would vaporize a city. Each reactor makes 250 kg of plutonium a year. It is postulated that less than one kilo of plutonium, if adequately distributed, could induce lung cancer in every person on earth.
Conclusion
In summary, the radioactive contamination and fallout from nuclear power plant accidents will have medical ramifications that will never cease, because the food will continue to concentrate the radioactive elements for hundreds to thousands of years. This will induce epidemics of cancer, leukemia and genetic disease. Already we are seeing such pathology and abnormalities in birds and insects, and because they reproduce very fast it is possible to observe disease caused by radiation over many generations within a relatively short space of time.
Pioneering research conducted by Dr Tim Mousseau, an evolutionary biologist, has demonstrated high rates of tumors, cataracts, genetic mutations, sterility and reduced brain size amongst birds in the exclusion zones of both Chernobyl and Fukushima. What happens to animals will happen to human beings. [7]
The Japanese government is desperately trying to “clean up” radioactive contamination. But in reality all that can be done is collect it, place it in containers and transfer it to another location. It cannot be made neutral and it cannot be prevented from spreading in the future. Some contractors have allowed their workers to empty radioactive debris, soil and leaves into streams and other illegal places. The main question becomes: Where can they place the contaminated material to be stored safely away from the environment for thousands of years? There is no safe place in Japan for this to happen, let alone to store thousands of tons of high level radioactive waste which rests precariously at the 54 Japanese nuclear reactors.
Last but not least, Australian uranium fuelled the Fukushima reactors. Australia exports uranium for use in nuclear power plants to 12 countries, including the US, Japan, France, Britain, Finland, Sweden, South Korea, China, Belgium, Spain, Canada and Taiwan. 270,000 metric tons of deadly radioactive waste exists in the world today, with 12,000 metric tons being added yearly. (Each reactor manufactures 30 tons per year and there are over 400 reactors globally.)
This high-level waste must be isolated from the environment for one million years – but no container lasts longer than 100 years. The isotopes will inevitably leak, contaminating the food chain, inducing epidemics of cancer, leukemia, congenital deformities and genetic diseases for the rest of time.
This, then, is the legacy we leave to future generations so that we can turn on our lights and computers or make nuclear weapons. It was Einstein who said “the splitting of the atom changed everything save mans’ mode of thinking, thus we drift towards unparalleled catastrophe.”
The question now is: Have we, the human species, the ability to mature psychologically in time to avert these catastrophes, or, is it in fact, too late?
Disclaimer: The views, opinions and perspectives presented in this article are those of the author alone and does not reflect the views of the Australian Medical Student Journal. The accuracy, completeness and validity of any statements made within this article are not guaranteed. We accept no liability for any errors or omissions.
References
[1] Caldicott H. Helen Caldicott Foundation’s Fukushima Symposium. 2013; Available from: http://www.helencaldicott.com/2012/12/helen-caldicott-foundations-fukushima-symposium/.
[2] Japan sat on U.S. radiation maps showing immediate fallout from nuke crisis. The Japan Times. 2012.
[3] Bagge E, Bjelle A, Eden S, Svanborg A. Osteoarthritis in the elderly: clinical and radiological findings in 79 and 85 year olds. Ann Rheum Dis. 1991;50(8):535-9. Epub 1991/08/01.
[4] Tests find cesium 172 times the limit in Miyagi Yacon tea. The Asahi Shimbun. 2012.
[5] Yablokov AV, Nesterenko VB, Nesterenko AV, Sherman-Nevinger JD. Chernobyl: Consequences of the Catastrophe for People and the Environment: Wiley. com; 2010.
[6] Fukushima Health Management. Proceedings of the 11th Prefectural Oversight Committee Meeting for Fukushima Health Management Survey. Fukushima, Japan2013.
[7] Møller AP, Mousseau TA. The effects of low-dose radiation: Soviet science, the nuclear industry – and independence? Significance. 2013;10(1):14-9.
Originally published: http://www.amsj.org/archives/3487
http://www.helencaldicott.com/the-impact-of-the-nuclear-crisis-on-global-health/
May 29, 2017
Posted by dunrenard |
Fukushima 2017 | cesium 137, Fukushima, Iodine-131, plutonium, Radioactivity, Radionuclides, Strontium-90, Tritium |
1 Comment
Here we determined the 90Sr concentrations in the teeth of cattle abandoned in the evacuation area of the Fukushima-Daiichi Nuclear Power Plant (FNPP) accident. 90Sr activity concentrations in the teeth varied from 6–831 mBq (g Ca)−1 and exhibited a positive relationship with the degree of radioactive contamination that the cattle experienced. Even within an individual animal, the specific activity of 90Sr (Bq (g Sr)−1) varied depending on the development stage of the teeth during the FNPP accident: teeth that were early in development exhibited high 90Sr specific activities, while teeth that were late in development exhibited low specific activities. These findings demonstrate that 90Sr is incorporated into the teeth during tooth development; thus, tooth 90Sr activity concentrations reflect environmental 90Sr levels during tooth formation. Assessment of 90Sr in teeth could provide useful information about internal exposure to 90Sr radiation and allow for the measurement of time-course changes in the degree of environmental 90Sr pollution.
Introduction
The Fukushima-Daiichi Nuclear Power Plant (FNPP) accident released a substantial amount of radioactive nuclides into the atmosphere and caused extensive contamination of the environment1,2,3,4,5. The radioactivity of the typical fission products was estimated to be 8.2 PBq for 137Cs, 9.8 PBq for 134Cs, and 0.14 PBq for 90Sr2,6. In June 2011, the Japan Ministry of Education, Culture, Sports, Science, and Technology (MEXT) reported that 0.1−6 kBq m−2 of 90Sr and 0.3−17 kBq m−2 of 89Sr were detected in the soil of areas within a 20-km radius from the FNPP (i.e., the former Fukushima evacuation zone)7. As 89Sr has a relatively short half-life of 50.5 days, its presence suggests that these radionuclides did not originate from global fallout due to nuclear weapons testing, but from the FNPP accident.
The long half-life (28.8 y) and bone-seeking properties of 90Sr make it a concerning artificial radionuclide among the fission products found near FNPP. Although radioiodine and caesium are more noticeable in quantity, 90Sr can persist in bone with a retention half-life of over 10 years, depending on bone type8,9,10,11. Moreover, its daughter nuclide, 90Y, emits β-rays (2.28 MeV) that may have adverse effects on the bone marrow. Thus, some attention has been paid to the determination of 90Sr content in bone and particularly in teeth. Sr is incorporated into the tooth during calcification. Once incorporated, it remains in enamel and dentine until the tooth falls out or is extracted12. Therefore, 90Sr activity concentration in a given tooth is a reflection of environmental 90Sr contamination levels when the tooth was formed.
Several studies have taken advantage of this phenomenon to understand the long-term effects of nuclear activity on humans. For example, 90Sr incorporation into human teeth has been observed after the Techa River region was contaminated by the release of liquid radioactive waste into the river during the early 1950s8,13,14,15. Similarly, the deciduous teeth of Swiss children born between 1952 and 2002 exhibited 90Sr activity concentrations that correlated with atmospheric rises in 90Sr levels, which resulted from nuclear weapons testing during that period11. Increases in tooth 90Sr activity concentrations following the 1986 Chernobyl accident have also been reported11,16,17. These observations indicate that 90Sr activity concentration in teeth is an effective indicator of 90Sr contamination levels in the environment. However, while studies have examined 90Sr contamination in soil, vegetation, the nearby seawater and fish after the FNPP accident18,19,20,21,22,23,24, no studies to date have reported on 90Sr activity concentrations in teeth or bones. We thus have little direct data on how much FNPP-related contamination affected animals, which is essential for fully understanding the extent of environmental pollution in the area.
In the aftermath of the FNPP accident, thousands of cattle were abandoned in the evacuation zone. These cattle subsisted on natural food and water in the contaminated environment. Previously, we investigated the activities of 134Cs, 137Cs, 110mAg, and 129mTe in cattle within a 20-km radius around the FNPP, and demonstrated that radioactive Cs concentrations in organs and plasma were dependent on the feeding conditions and the geographic location of the cattle25. We also separately examined the effect of radioactive Cs on cattle testes after the FNPP accident26. We now expand on these studies by examining 90Sr concentrations in cattle teeth and relating them to other measures of environmental pollution.
Results
Activity concentration of 90Sr in the soil of cattle residence areas
Figure 1 details the locations of cattle residence after the FNPP accident. Areas H and L are situated in the government-delineated evacuation zone, and their 90Sr activity concentrations in soil are 94–1500 Bq m−2 (average: 738 Bq m−2) and 39–380 Bq m−2 (average: 195 Bq m−2), respectively7. We chose area C in Iwate Prefecture as the control region because it is approximately 250 km north of the nuclear plant and is considered free from FNPP-related 90Sr pollution. The activity concentration of 90Sr in soil in area C is 95–99 Bq m−2 (average: 96 Bq m−2)27.

FNPP: The Fukushima-Daiichi Nuclear Power Plant. H: High-contamination area (10–30 μSv h−1), 5 km west of FNPP. L: Low-contamination area (0.8–1.2 μSv h−1), 16 km south-west of FNPP. Areas H and L were in the evacuation zone. C: Control area in Iwate Prefecture, 250 km north of FNPP. The maps were modified from open-access base maps freely available for public and academic use (source: http://maps.gsi.go.jp, from the Geographic Information Authority of Japan).
adioactivity of 90Sr in cattle teeth
- 90Sr activity concentration
Figure 2 summarizes the 90Sr activity concentrations (90Sr activity/amount of Ca) in various teeth (deciduous molars, premolars, and molars (Supplementary Table S1)).
We detected 90Sr in all examined teeth. Activity concentrations varied significantly with area (p = 0.0000, Kruskal-Wallis test): high 90Sr concentrations (61–831 mBq (g Ca)−1) were observed in area H, mid-range concentrations (22–311 mBq (g Ca)−1 were observed in area L, and the lowest concentrations (6–35 mBq (g Ca)−1) were observed in control area C. These 90Sr activity concentrations in teeth were significantly correlated with 90Sr concentrations in the soil of areas H, L, and C (ρ = 0.8441, p < 0.01, Spearman’s rank-order correlation analysis).
- Specific activity of 90Sr
Figure 3 shows the specific activities of 90Sr (90Sr radioactivity/amount of stable Sr) in teeth (Supplementary Table S2). 90Sr specific activities were similar to the 90Sr activity concentration trends: higher (214–1351 Bq (g Sr)−1) and lower (60–641 Bq (g Sr)−1) activity were observed for areas H and L, respectively. The lowest specific activity (13–78 Bq (g Sr)−1) was observed in teeth from control area C. The specific activity differed significantly across areas H, L, and C (p = 0.0000, Kruskal-Wallis test). Moreover, specific activity was significantly correlated with 90Sr concentrations in the soil of areas H, L, and C (ρ = 0.8507, p < 0.01, Spearman’s rank-order correlation analysis).
- Activity concentrations and specific activities of 90Sr in teeth at different developmental stages.
The four young cattle examined in this study (H-young-1, H-young-2, L-young-1, and L-young-2) were 8 months old when the FNPP accident occurred (Table 1) and their molars ranged across developmental stages: development of the deciduous molars (DM1, DM2, and DM3) were either complete or in the late stage, the molars (M1, M2, and M3) were actively developing, and the premolars (P1, P2, and P3) were still early in development28 (Fig. 4a). 90Sr activity concentrations and specific activities were low in deciduous molars, higher in molars, and highest in premolars for each individual (p = 0.0006 and 0.0004 for 90Sr activity concentration and 90Sr specific activity, respectively; Kruskal-Wallis test; Figs 2 and 3).
We also determined the activity concentrations and specific activity of 90Sr in the teeth of two adult cattle (L-adult-1 and L-adult-2) from area L, that were 22 and 51 months old, respectively, during the FNPP accident. Based on their age, we assumed that development of permanent molars and premolars were complete at that point (Fig. 4b). We found low levels of 90Sr activity concentrations (22–91 mBq (g Ca)−1) and specific activities (60–166 Bq (g Sr)−1) in all adult teeth, but in contrast to young cattle (L-young-1 and L-young-2), no significant differences existed across adult molars and premolars in both 90Sr activity concentration and specific activity (p = 0.6310 and 0.3367, respectively; Kruskal-Wallis test).
Concentration of stable Sr in cattle teeth
We compared stable Sr concentrations in teeth formed before and after the accident (Table 2).

We found that Sr concentrations in the teeth of young cattle in area H and L differed across the deciduous molars, molars, and premolars. The deciduous molars, fully developed before the accident, exhibited low Sr concentrations, while the premolars that developed post-accident exhibited high concentrations. Molars undergoing active development when the accident occurred exhibited mid-range values. The variation in Sr concentrations across teeth was statistically significant in young cattle from areas H and L (p = 0.0211, Kruskal-Wallis test). In contrast, the dentition of adult cattle from area L (molars and premolars; most deciduous molars had fallen out by the time of sampling, see Table 2) was already fully developed when the accident occurred and exhibited no differences in stable Sr concentration (p = 1.0000, Kruskal-Wallis test). We also found no differences among the deciduous molars, molars, and premolars of the young control cattle (p = 0.7488, Kruskal-Wallis test).
Discussion
The results of our study demonstrated that activity concentrations and specific activities of 90Sr in cattle teeth varied in accordance with the degree of 90Sr pollution in the cattle residence areas. After the FNPP accident, the cattle were released to the field and subsisted on grasses, leaves, and river or swamp water in the polluted environment. The contamination of natural food and water consumed by the cattle likely contributed to 90Sr activity differences we observed in the H-area teeth versus L-area teeth.
Patterns corroborating our results have been reported in cow teeth from 16 contaminated areas in the Mayak region of the former Soviet Union29. The study using imaging plates showed that 90Sr activity concentrations in the teeth were 0.09–2.96 kBq (g tissue)−1 on average and were positively correlated with soil contamination levels (<3.7–185 kBq m−2). Although the degree of 90Sr contamination in our study areas was much lower than contamination in the Mayak region, we note the similar relationship between environmental 90Sr and tooth 90Sr activity concentration: 90Sr in the teeth faithfully reflects the degree of 90Sr pollution in the environment when the tooth was formed.
Small amounts of 90Sr were detected in the teeth of control cattle. Similarly, low levels of 90Sr have been found elsewhere in Japan even before the accident occurred. For example, 90Sr activity concentrations in cattle bones from Hokkaido (located on the northern edge of Japan and relatively far from the FNPP) were approximately 72 mBq (g Ca)−1 in 199630 and 26 mBq (g Ca)−1 in 200831. Both concentrations are higher than the 90Sr activity concentrations in the control teeth (14 ± 7 mBq (g Ca)−1) of this study. Possible sources for pre-FNPP radioactivity in Hokkaido are either the Chernobyl accident or nuclear weapons testing. Although 90Sr fallout from Chernobyl had been detected in Japan previously, the amount was far less than fallout from nuclear weapons testing32. Moreover, increases to 90Sr activity concentrations in Hokkaido cattle bones were not observed at the time of the Chernobyl accident30. Therefore, the low levels of 90Sr activity measured in our controls probably stemmed from the atmospheric nuclear weapons testing conducted during the 1950s–1970s.
In this study, we took advantage of cattle tooth development to examine pre- and post-accident levels of 90Sr. Tooth development follows a fixed trajectory that varies across species. In cattle, deciduous molars first form during the prenatal period, followed by molars. Premolars then form during the early postnatal period, with the first premolars (P1) forming in the last stage of dentition, beginning from 12–18 months and completing at 18–24 months28. Therefore, cattle younger than 24 months old possess teeth across all dentition developmental stages. Moreover, teeth at early developmental stages during the accident would primarily form under a polluted environment, incorporating large amounts of 90Sr. In contrast, the formation of teeth at late developmental stages would be mostly complete during the accident, resulting in the incorporation of less 90Sr. Furthermore, 90Sr activities in the teeth of adult cattle were low (Fig. 2c) and nearly constant, although the adults had resided in area L, the same location as two of the young cattle.

DM: Deciduous molars (first, second, and third deciduous molar); M: Molars (first, second, and third molar); P: Premolars (first, second, and third premolar). Along the x-axis, the teeth are arranged according to the chronological order of tooth development (DM, M, P). Error bars represent the standard deviation.
We observed 90Sr activity even in teeth that had fully developed before the accident (i.e., deciduous molars of young cattle in areas H and L, as well as deciduous molars, molars, and premolars of adult cattle in area L). These levels were occasionally higher than levels in control cattle (compare Fig. 2c to Fig. 2d, and Fig. 3c to 3d), although essential incorporation of 90Sr was not expected during tooth development. These higher than expected concentrations may have been due to non-specific 90Sr adsorption on the tooth surface via contaminated food or water. Alternatively, they could have resulted from the deposition of dental calculus on the tooth surface after the FNPP accident. Furthermore, as described by Tolstykh et al.8,14, ion exchange between the dentine tubule and pulp surfaces with the secondary dentine formation in the pulp could proceed even after complete tooth development. These interactions may also contribute to the higher 90Sr activities in adult teeth from area L compared with control teeth.

DM: Deciduous molars (first, second, and third deciduous molar); M: Molars (first, second, and third molar); P: Premolars (first, second, and third premolar). Along the x-axis, the teeth are arranged according to the chronological order of tooth development (DM, M, P). Error bars represent the standard deviation.
Data on the stable Sr concentrations in teeth formed pre- and post-accident (Table 2) accorded with the radioactivity data. Specifically, in young cattle of both areas H and L, teeth formed before the accident contained less stable Sr than teeth formed after the accident. The teeth of adult cattle and control cattle, however, did not exhibit a pre- and post-accident difference in stable Sr. Again, similar to our findings for 90Sr specific activity, these patterns in the experimental young cattle are likely the result of differences between pre-accident farm feed and the post-accident natural resources that the cattle ingested. Although we did not measure Sr contents in the diets of our subject cattle, our data on stable Sr suggest that the natural grasses, leaves, and water ingested by the cattle likely contain more Sr than their former farm feed. These data suggest that stable Sr concentrations could be also used in conjunction with 90Sr specific activity as a metric for environmental Sr levels.
We have shown that 90Sr incorporation into teeth is cumulative during tooth development, reflecting the degree of environmental 90Sr contamination in that time. Unlike bone, the tooth has essentially no metabolism. Therefore, the 90Sr in tooth is a potentially useful indicator for estimating the internal radiation exposure of individuals affected by nuclear activity during their tooth formation periods. Furthermore, by measuring 90Sr activities in different teeth, we can take advantage of the various developmental trajectories of animal (including human) dentition and use the known chronologies of individual tooth growth to track time-course changes in the degree of environmental contamination.
Materials and Methods
Ethics
This study is one of the national projects associated with the Great East Japan Earthquake that occurred on March 11, 2011. All protocols were approved by the Tohoku University (No.2014KDO037). All methods detailed below were carried out in accordance with these guidelines.
On May 12, 2011, the Japanese Ministry of Agriculture Forestry and Fisheries (MAFF) ordered euthanasia of cattle abandoned in the evacuation zone to prevent radio-contaminated beef from entering consumer products25. Euthanasia was carried out by veterinarians belonging to the Livestock Hygiene Service Center (LHSC) of Fukushima Prefecture, in accordance with the Ethical Regulations for Animal Experiments and Related Activities at MAFF. These regulations are based on the June 2007 euthanasia guidelines issued by the American Veterinary Medical Association. The cattle were anesthetized with an intramuscular injection of xylazine hydrochloride (0.2 mg kg−1). They were then euthanized via an overdose of intravenous sodium pentobarbital (20 mg kg−1), followed by intravenous suxamethonium hydrochloride (2 mg kg−1). Before performing euthanasia, veterinarians obtained informed consent from the livestock owners, who were identified from the cattle ear tags. We collected organs and tissues, including mandibular bones, from euthanized cattle with the help of LHSC veterinarians.
Collection of tooth samples
We selected six cattle (H-young-1, H-young-2, L-young-1, L-young-2, L-adult-1, and L-adult-2) residing in the two FNPP evacuation areas (H and L; Fig. 1) for the current study. Two other cattle (control-1 and control-2) from the uncontaminated area C (Fig. 1) were chosen as controls. The mandibular bones of control cattle, including teeth, were supplied by the Iwate Chikusan Ryutsu Center Co., Ltd. in Iwate Prefecture. The characteristics of the study subjects are summarized in Table 2.
Mandibular bones were dissected from the cattle skulls and radiographs were taken with X-ray equipment (Panoramic Radiograph, Auto-IIIE, Asahi Roentgen Ind. Co., Ltd.) to classify the developmental stages of molar dentition. The deciduous molars, molars, and premolars were then dissected from the mandible and kept in 70% ethanol until needed. We air-dried teeth in a desiccator after removing any surface debris with toothbrushes and dental scalars. The tooth was then crushed with a hammer and powdered using a tissue lyser (TissueLyser II, Qiagen Co., Ltd.). The powdered teeth (1.5 g) were then incinerated in a muffle furnace at 450 °C for 12 h and used for 90Sr, stable Sr, and Ca measurements, as described in the following subsection.
In this study, we used the entire tooth for analysis, without separating enamel and dentine. The cattle molar is classified as a hypsodont tooth (drycodont), morphologically characterized by extremely high and long crowns that occupy more than 4/5th of the whole tooth33. The crown elongates parallel to the growing axis and consists of both enamel and dentine. The latter is metabolically inert, like the enamel, and therefore accumulates Sr in the same way. Additionally, Sr concentration in dentine is slightly higher than in enamel, but the difference is small (70–620 in dentine versus 25–600 μg g−1 in enamel34). Because both tissues develop almost simultaneously during crown formation, and because the hypsodont tooth primarily consists of the crown, we thought that the relationship between molar development and 90Sr accumulation could be properly assessed even without separating enamel and dentine. Further, using the whole tooth allows us to track changes in 90Sr deposition over a longer period, because complete tooth formation takes over 2 years, whereas separate parts of the tooth take less time to develop.
Chemical separation of 90Sr in the teeth
Many methods have been developed for the separation of Sr from large amounts of Ca, including co-precipitation, liquid-to-liquid extraction, ion-exchange or extraction chromatography, and combinations of these techniques24,35,36. For this study, we chose the fuming nitric acid method. Although newer methods are less demanding and occasionally result in higher yields, the use of fuming nitric acid remains reliable and robust under conditions of large sample amounts and extremely high quantities of co-existing Ca35, which was the case here.
We dissolved 1 g of the incinerated sample in 10 mL of 60% HNO3 (analytical grade, Kanto Chemical Co., Inc.), and added 20 mg of Sr2+ carrier to the solution. We then added 10 mL of fuming nitric acid (analytical grade, Kanto Chemical Co., Inc.) to precipitate Sr(NO3)2. We removed the remaining Ca by dissolving the precipitate in 10 mL of distilled water, and again adding 10 mL of fuming nitric acid. The resultant supernatant was discarded. By repeating this procedure two to three times, the Sr within each sample was successfully separated from Ca as Sr (NO3)2. Sr (NO3)2 was dissolved in 10 mL of distilled water to form Solution A, which was subjected to further chemical separation from Ra and Pb.
Trace amounts of natural 226Ra (half-life: 1600 y), 228Ra (half-life: 5.75 y) and 210Pb (half-life: 22.3 y) are present in teeth35. These radionuclides interfere with accurate β-ray measurement of 90Sr. Therefore, these radionuclides were removed via co-precipitation with BaCrO4 using the following procedure.
First, 2 mL of acetate buffer solution was added to Solution A. Then, 10 mg of Ba2+ was added, followed by dilution with distilled water to a volume of 20 mL. Ra and Pb were scavenged with BaCrO4 precipitate, formed by adding 0.1 mL of 1.5 M Na2CrO4 solution. After centrifugation, the supernatant containing Sr was separated. To the supernatant, 1 mL of concentrated NH4OH and 2 mL of saturated (NH4)2CO3 solution were added to precipitate SrCO3. Lastly, the precipitate was dissolved in 10 mL of 1 M HNO3 (Solution B).
To distinguish the growth curve of 90Y from 90Sr during β-ray measurement, 90Y was removed by co-precipitation with Fe (OH)3. Fe3+ (2 mg) was added to 10 mL of Solution B. Concentrated NH4OH was then added until Solution B’s pH was 8–9. This step precipitated Fe(OH)3, which was used to scavenge 90Y. The supernatant was filtered from the precipitate using a glass microfiber filter (Whatman GF/F 25 mm, GE Healthcare Life Science Co.). Finally, Sr in the supernatant was precipitated as SrCO3 by adding 3 mL of saturated (NH4)2CO3 solution. The precipitate was filtrated with a membrane filter (JAWP02500, 25 mm diameter, Merck Millipore Co.), and stored in a stainless-steel sample dish (E0802001, 25 mm, 6 mm height, Chiyoda Technology Co.). The dish was covered with polyimide film (7.5 μm thick) to avoid further contamination and submitted for β-ray measurement.
Chemical yields of Sr, or the recovery of Sr carrier added to the sample solution at the beginning of the separation procedure, were 70% on average, with a range of 50–96% for 114 determinations.
β-ray measurement
The β-rays emitted from 90Sr and its daughter 90Y were measured with a low background gas flow counter (LBC-4201B, Hitachi-Aloka Medical, Ltd.) for 3–12 h.
Following chemical separation of Sr, we monitored the growth of 90Y from 90Sr in SrCO3 precipitate. We measured β-rays 5 to 6 times within a fortnight of the separation. Using these measurements of radioactivity, we created a time-course plot to check whether the increase in β-ray counts fits the theoretical growth curve of 90Y. 90Y growth typically reached secular equilibrium with 90Sr 2 weeks after Sr separation, upon which we measured total radioactivity from 90Y and 90Sr. To correct for the self-absorption of β-rays by the measured sample, we used an absorption coefficient that was experimentally obtained with β-rays from known amounts of 90Sr and 90Y against the thickness of SrCO3 precipitate.
The background level of the gas-flow counter used in this study was 0.155 ± 0.015 cpm (3σ: 0.044 cpm) per 12 hr. Measurement efficiency was 0.339 ± 0.001 when the 90Sr standard sample was 88.4 Bq with a thickness of 24 mg cm−2. Assuming that the recovery of chemical separation of Sr is 70%, the detection limit of 90Sr in 1 g of incinerated sample is 4.94 mBq (g Ca)−1. All values obtained in this study were above this detection limit.
90Sr activity due to decay was corrected to March 11, 2011 (the day of the FNPP accident).
Determination of stable Ca and Sr in the teeth
We determined Ca and Sr content in teeth using the inductively coupled plasma atomic emission spectrometer (ICP-AES; ICPE-9000, Shimadzu Co., Ltd.) at the Research and Analytical Center for Giant Molecules, Graduate School of Science, Tohoku University. Incinerated teeth samples were dissolved in 60% HNO3, and a portion of the solution was diluted 10,000 times for the measurements. Sr was determined using a standard addition method with a wavelength of 407.771 nm, and Ca was determined using a calibration-curve correction method with a wavelength of 317.933 nm. Each measurement was performed in triplicate and the resultant values were averaged.

(A) H-young-2: Deciduous molars were fully developed. Of the molars, the root of the third molar was under active development. The premolars under the deciduous molars were in the early developmental stage. (A) L-adult-2: Both molars and premolars were fully developed. Permanent dentition was complete. DM1: First deciduous molar; DM2: Second deciduous molar; DM3: Third deciduous molar; M1: First molar; M2: Second molar; M3: Third molar; P1: First premolar; P2: Second premolar; P3: Third premolar.
Statistical analysis
Because the data were non-parametric, we chose Kruskal-Wallis tests for our analyses, with significance set at p < 0.05. Tests were one-tailed. Data on DM1, DM2, and DM3 were grouped together as deciduous molars (N = 16); data on M1, M2 and M3 were grouped as molars (N = 24); and data on P1, P2, and P3 were grouped as premolars (N = 21). Independent variables used in the test were area (H, L, C) and type of tooth (deciduous molars, molars, premolars). Dependent variables were 90Sr activity concentrations, 90Sr specific activity, and stable Sr concentrations. We used Spearman’s rank-order correlation analysis to look for significant correlations between 90Sr radioactivity in the soil and in the teeth. All analyses were performed in STATISTICA (Ver. 06J, StatSoft Co., Ltd.).
Source: Koarai, K. et al.90Sr in teeth of cattle abandoned in evacuation zone: Record of pollution from the Fukushima-Daiichi Nuclear Power Plant accident. Sci. Rep. 6, 24077; doi: 10.1038/srep24077 (2016).
http://www.nature.com/articles/srep24077
April 12, 2016
Posted by dunrenard |
Fukushima 2016 | Contamination, Fukushima continuing, radiation, Strontium-90 |
1 Comment

Dogs fed sardines show high Strontium levels
by Dr. Peter Dobias, DVM
Why you might want to cut out small fish from your dog’s diet
I have had two dog patients with severely elevated levels of the element strontium. The interesting part is that these two dogs were fed a high amount of sardines and I highly suspect that strontium is coming from this source.
Strontium acts in the body the same way as calcium and deposits in bones. Sardines and other small fish are eaten whole with the bones and that is why they are more likely a source of this toxic element.
The reason why I am concerned is that the radioactive isotope strontium 90 is a toxic carcinogen and it has been released in Japan’s Fukushima disaster.
Here is an example of the results:
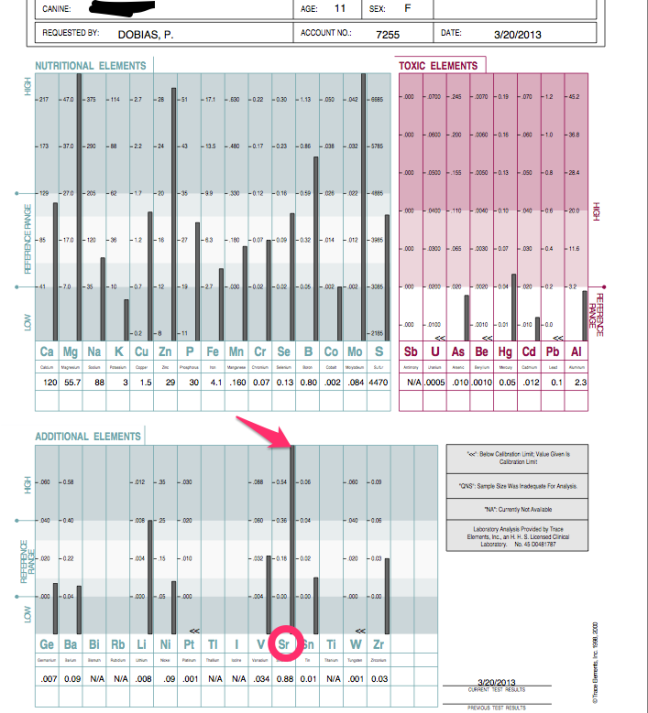
As a veterinarian, I source from almost three decades of experience, but still I like to see the proof. Hair testing for minerals and toxic elements has been really helpful because it is highly accurate and shows what is happening in different groups of dogs.
In the course of many years of testing, I have learned that dogs who eat fish-based foods have elevated mercury levels and sardines appear to be the cause of increased strontium. Since the Fukushima nuclear accident strontium is continuously being released into the oceans and not much is being done to inform the general public.
Sadly, I have noticed that dogs who have epilepsy have higher than average levels of strontium and mercury, which made me recommend against feeding fish and sardines to dogs, despite their nutritional benefits. Fish is not what it used to be.
http://peterdobias.com/blogs/blog/11014105-dogs-fed-sardines-show-high-strontium-levels
http://peterdobias.com/blogs/blog/113943301-how-to-avoid-foods-high-in-arsenic-and-keep-your-dog-healthy?utm_source=facebook&utm_medium=cpc&utm_campaign=Content
March 31, 2016
Posted by dunrenard |
Fukushima 2016 | Contaminated Foods, Contamination, Fukushima Daiichi, Sardines, Strontium-90 |
1 Comment

試料 名 Sample:
野生サーモン-シロザケ-メス
(フリーズドライ)
Wild Chum Salmon – female
(Freeze Dried)

採取 場所 Origin: Kilby Park, BC Canada
採取年月 Sampling date: 2014 年11月30日 (November 30, 2014)
測定日時 Date Tested : 2015 年3月31 日 (March 31, 2015)
測定時間 Duration : 57,600 秒(seconds)

試料容器 Container: 500mLマリ ネリ容器(Marinelli)
試料重量 Sample weight: 144.2g
乾燥前 Before dehydration: 2010g → 700g
(Citizen Radioactivity Measuring Station, Shinjyuku-Yoyogi) with Germanium Detector
March 12, 2016
Posted by dunrenard |
Canada | Contamination, Fish, Strontium-90 |
Leave a comment

A movement calling on people to retain their children’s baby teeth to help study radiation exposure in the wake of the Fukushima nuclear disaster is gaining momentum in Japan.
The radioactive material strontium-90 is easily absorbed into baby teeth, and last year a group of experts formed the “Preserving Deciduous Teeth Network (PDTN),” urging people to keep their children’s baby teeth.
“Baby teeth are evidence of exposure to radiation. We urge people to keep them for the future,” says Takemasa Fujino, 67, a joint head of the network.
Fujino is president of a medical institution that operates three dental clinics in the Tokyo metropolitan region. One clinic is in Matsudo, Chiba Prefecture, which was regarded as a “hot spot” with relatively high levels of radiation following the March 2011 outbreak of the Fukushima No. 1 Nuclear Power Plant disaster.
With residents feeling uneasy, in 2011 Fujino began calling for people to preserve their children’s baby teeth, wanting to do something as a dentist to protect children’s lives and health.
So far, Fujino has had about 500 baby teeth donated, and has commissioned a Swiss testing facility to analyze some of them. Next year the network plans to establish its own testing facility in central Japan.
Baby teeth are formed from when the child is in the womb. “The teeth of children that were fetuses five years ago at the time of the accident will be coming at about this point exactly, and the movement to preserve them will become even more important,” Fujino says.
http://mainichi.jp/english/articles/20160307/p2a/00m/0na/015000c
March 7, 2016
Posted by dunrenard |
Fukushima 2016 | Babyteeth, Contamination, Disaster, Fukushima continuing, Strontium-90 |
Leave a comment

From Tepco’s report released on 9/4/2015, the density of Strontium-90 increased to 155% of the previous highest reading in the seaside of Reactor 2.
It was measured in groundwater gathered to pump up. The sampling date
was 8/3/2015, Sr-90 density was 2,800,000 Bq/m3. The previous highest reading was 1,800,000 Bq/m3, which was analyzed on 7/2/2015.
From the report of 9/15/2015 about the same area, the density of Mn-54, which has 310 days of a half-life, reached the highest reading of 680 Bq/m3 on 9/10/2015. 4 days later, it rose up to 970 Bq/m3 again.
http://www.tepco.co.jp/nu/fukushima-np/f1/smp/2015/images/2tb-east_15090401-j.pdf
http://www.tepco.co.jp/nu/fukushima-np/f1/smp/2015/images/2tb-east_15091501-j.pdf
Source: Fukushima Diary
Sr-90 density rose up 155 percent of the previous highest reading in the seaside of Reactor 2
September 18, 2015
Posted by dunrenard |
Japan | Fukushima Daiichi, reactor 2, Strontium-90 |
Leave a comment

From Tepco’s report published on 7/17/2015, Strontium-90 density in seawater of Fukushima plant port became the highest since they started measuring.
The sampling location was between water intake of Reactor 3 and 4, and also screen of Reactor 4, which are in front of Reactor 3 & 4.
The samples were collected on 6/1/2015. This analysis result has not been published for over a month.
1,500,000 Bq/m3 of Strontium-90 was detected from both of the samples.
The previous highest reading was 1,000,000 Bq/m3, which was detected at the same sampling location on 5/4/2015.
http://www.tepco.co.jp/nu/fukushima-np/f1/smp/2015/images/2tb-east_15071701-j.pdf
Source: Fukushima Diary
Highest Strontium-90 density detected in seawater of Fukushima plant port / 1,500,000 Bq/m3
July 19, 2015
Posted by dunrenard |
Japan | Fukushima Daiichi, Strontium-90 |
Leave a comment

On 6/19/2015, Tepco announced they measured 1,000,000 Bq/m3 of Strontium-90 at two locations in Fukushima plant port.
This is the highest reading in recorded history. The sample is the port seawater. Sampling date was 5/4/2015.
The location was near the water intake of Reactor 3 and 4, and also the screen of Reactor 4.
The previous highest readings were lower than 700,000 Bq/m3.
Tepco has not made any announcement on this rapid increase.
http://www.tepco.co.jp/nu/fukushima-np/f1/smp/2015/images/2tb-east_15061901-j.pdf
Source: Fukushima Daiichi
1,000,000 Bq/m3 of Sr-90 detected in seawater of Fukushima plant port / Highest in recorded history
June 22, 2015
Posted by dunrenard |
Japan | Fukushima Daiichi, Radiation Levels, Strontium-90 |
Leave a comment

January 27, 2015
On 1/27/2015, Tepco announced they measured high density of Strontium-90 from groundwater in the seaside of Reactor 2.
It was 31,000,000 Bq/m3. The sampling point was the boring well, which is the closest to Reactor 2.
This is the highest density measured from this boring well, which is 10% more than the previous highest record.
The sampling date was last December. No Sr-90 data of January has been published.
http://www.tepco.co.jp/nu/fukushima-np/f1/smp/2015/images/2tb-east_15012701-j.pdf
Source: Fukushima Daiichi
31,000,000 Bq/m3 of Strontium-90 measured at the nearest boring well to Reactor 2
January 29, 2015
Posted by dunrenard |
Japan | Fukushima Daiichi, Strontium-90 |
Leave a comment
October 20, 2014
2,800 ~ 11,000 Bq/m3 of Strontium-90 have been detected from pumped water around Reactor 1 and 3, according to Tepco.
On 10/1/2014, Tepco released nuclide analysis data of groundwater. The tested groundwater was pumped up from the facilities called “sub-drain” located beside Reactor 1 ~ 4.
These “sub-drains” were originally to reduce groundwater volume to flow into the basement of each reactor building, however abandoned because of the high level of contamination after 311. Tepco is trying to restart using these sub-drains to pump up highly contaminated water and to discharge to the sea.
(cf, Tepco to pump up highly contaminated groundwater for potential discharge today / Drainage plan submitted to NRA.
The samples were taken this September and last September. From the sample near Reactor 1, 11,000 Bq/m3 of Strontium-90 was detected last September. From the sample near Reactor 3, 2,800 Bq/m3 of Strontium-90 was measured this September.
These readings were not checked by third party organizations, so the actual density can be higher than announced.
Either way, the data shows groundwater contamination is spreading from around the reactor buildings to the outside of the port. (cf, Strontium-90 detected outside of Fukushima port / Highest reading in front of Reactor 4 too.
Click to access around_rb_141001-j.pdf
Source: Fukushimary Diary
Strontium-90 detected in potentially discharged water from Reactor 1 and 3
October 20, 2014
Posted by dunrenard |
Japan | Discharged Water, Fukushima Daiichi, Reactor1, Reactor3, Strontium-90 |
Leave a comment